The Effects of Dietary
Molybdenum Sulfur
and Iron on Absorption of Three Organic Copper Sources
H. D. Ashmead
S. D. Ashmead
Albion Advanced Nutrition, Clearfield, Utah, U.S.A.
KEY WORDS: Molybdenum, copper, feed, steer, nutrition, veterinary.
Abstract
Twenty-seven Angus steers were fed identical diets for
120 days fortified with 10 mg copper (Cu)/kg feed from either Cu amino
acid chelate, Cu proteinate, or Cu amino acid complex plus 750 mg Fe/kg
feed, 0.35% S, and 10 mg Mo/kg feed, which are known Cu absorption/metabolism
antagonists. Analysis of liver, blood serum and ceruloplasmin indicated
that while all 3 sources of Cu were initially affected by the dietary
antagonists, only the amino acid chelated source was able to overcome
these effects and provide significantly more available Cu to the animals.
Introduction
When molybdenum intake exceeds 1 mg/kg of dry matter,
a reduction in copper (Cu) absorption in the ruminant usually results.1,2
This antagonism is exacerbated by the presence of as little as 0.2%
sulfur (S) as a sulfide ion. The Mo/S synergistic effect begins with
the substitution of S for oxygen in the MoO42–
ion to ultimately yield tetrathiomolybdate (MoS42–):
MoO4 2– Æ MoO3S2– Æ MoO2S22– Æ MoOS32– Æ MoS42–
MoS42–
has the potential to bind ruminal Cu ions, rendering them nutritionally
unavailable to the animal. When Mo intake exceeds 10 mg/kg of dry matter,
the MoS42–
formed may also interfere with Cu metabolism because of the formation
of highly stable CuMoS4
molecules in the plasma (Figure 1).3,4
As little as 250 mg iron (Fe)/kg feed can reduce Cu reserves
in ruminant tissues.1,5 While the exact inhibition mechanism is not
completely elucidated, it is believed that ionic Fe and Cu may compete
for the same luminal binding proteins necessary for mucosal transport
with Fe being preferentially bound.6–8 The binding of Fe2+ with ruminal
S2– may also result in an insoluble FeS compound. The S2– is donated
by the Cu binding transport proteins in the mucosa which are rich in
sulfhydryl groups. With the loss of S2–, the formation of the mercaptide
bonds necessary to complex potentially available Cu ions is blocked.9
Regardless of the exact mechanisms involved, the fact
remains that when Mo, S, and Fe are included in the ruminant feed at
minimum concentrations of 1 mg/kg, 0.2%, and 250 mg/kg, respectively,
dietary ionic Cu absorption is reduced.
While chemically different, copper amino acid complex,
copper proteinate, and copper amino acid chelate are usually grouped
together as “organic” minerals and are frequently but erroneously thought
of as having similar bioavailabilities. Copper amino acid complex is
defined by the Association of American Feed Control Officials (AAFCO)
as the product resulting from the complexing of a soluble copper salt
with an amino acid.10 Cu proteinate is defined by AAFCO as the product
resulting from the chelation of Cu ions to amino acids and/or partially
hydrolyzed protein.10 AAFCO has defined Cu amino acid chelate as the
chelation of ionic Cu to one to three amino acids, but unlike Cu proteinate,
not to partially hydrolyzed protein.10 If Cu is chelated into either
the proteinate or amino acid chelate molecule, the Cu must be bonded
to the ligand at two sites by coordinate covalent bonds thus forming
at least one heterocyclic ring. The strength of the Cu chelate and consequently
the degree of potential Cu reactivity with other complexes in the rumen
will vary with the size of the chelating ligand, the stereochemistry
of the Cu chelate, the number of chelating ligands the ionic Cu is bonded
to, the size of those chelate rings formed with the single Cu ion, and
finally the basic strength of the chelating ligand.11
Cu proteinate, Cu amino acid complex, and Cu amino acid
chelate are all reported to be more bioavailable than commonly fed Cu
salts.12 In the case of either the complex or proteinate source of Cu,
it is believed that the Cu is protected from some chemical reactions
within the gastrointestinal tract, but their exact absorption mechanisms
are unknown.13 Several studies suggest that the greater bioavailability
of the copper amino acid chelate used in this study results from copper
protection in the gut followed by absorption of the intact molecule
into the mucosal cells where hydrolyzation subsequently occurs.7,14,15
If chelating or complexing Cu reduces its potential to react with ruminal
anions, then depending on the stability of that chelate or complex,
the anions that are antagonistic to dietary Cu may not have as great
of an effect on Cu absorption from these sources. The purpose of this
study was to determine if a Cu amino acid complex, a Cu proteinate source
and/or a Cu amino acid chelate was capable of protecting the Cu and
allowing its increased absorption in the presence of dietary Mo, S,
and Fe antagonists.
Materials
and Methods
Twenty-seven Angus steers of similar age with a mean weight
of 700 lbs (318 kg) were purchased from the same farm and each assigned
a number which was recorded on each animal’s ear tag. Following acquisition,
all of the animals were allowed to acclimate in a common pen for 14
days. Pelletized feeds were gradually introduced into their diets during
acclimation in order to allow them to become accustomed to the pelleted
form of treatments that would follow. At the conclusion of the adaptation
period (following the procedure of Hickok et al.,16 which eliminates
variability), a 0.5 g (net weight) liver biopsy was obtained from each
animal using a Tru-Cut® biopsy needle (Cardinal Healthcare, McGraw Park,
IL). Larry Hortsman, DVM, a faculty veterinarian at Purdue University
who is experienced in taking bovine liver biopsies, collected all the
samples during the study. The biopsies were removed from the caudal
region of the liver between the 12th and the 13th rib, 20 cm ventral
to the midline. The caudal region of the liver contains a higher amount
of Cu than other portions of the liver.16
Each
hepatic sample was washed with saline solution for 1 minute to remove
excess blood, blotted, and placed in a special sealed container identified
by the animal’s ear tag number. It was subsequently shipped cold by
overnight delivery to a certified analytical laboratory (Albion Laboratories,
Clearfield, Utah) where it was weighed both wet and dry. Each sample
was then liquefied in a Waring blender and subsequently digested in
concentrated nitric acid before being assayed for Cu, Fe, and Mo by
a plasma emission spectrophotometer equipped with an ultrasonic nebulizer.17,18
Concurrent to taking liver biopsies, blood was obtained
by venipuncture from each animal, spun, and the serum divided into two
parts, both of which were put in individual vacutainers designed for
serum storage. Each vacutainer was identified by the animal’s ear tag
number. A 0.5 mL sample was sent to the same analytical laboratory that
assayed the liver samples and was also assayed for Cu, Fe, and Mo using
plasma emission spectrophotometry.18,19 The second 0.5 mL serum sample
was sent to the laboratory of Robert DiSilvestro, PhD, Ohio State University,
where that sample was assayed for ceruloplasmin activity.19
Both laboratories
and all personnel were kept blind as to which animals received which
feed supplements as described below. Each liver and serum sample was
identified only by the number which corresponded to the ear tag of the
animal donating the samples.
Based on these pretrial assays, the cattle were distributed
into 3 pens of 9 steers each such that all initial Cu measurements were
more statistically equivalent. The statistical blocking for pen assignment
was based first on ceruloplasmin activity, second on serum Cu levels,
and finally on hepatic Cu levels.
Each pen was color-coded and randomly assigned a corresponding
color-coded treatment, described below. The color marking on the pen
matched the color of the feed tag of one of 3 basal diets. The herdsman
was blinded as to what the treatments contained, but simply fed each
group the correct amount of pelleted basal diet from the bag with a
feed tag color that matched the assigned color of the pen.
Except for the sources of Cu, all of the steers received
the same basal diet. The basal diet was divided into 3 parts, identified
as test pellet No. 1, test pellet No. 2, or test pellet No. 3. The basal
diet contained 17% crude protein, 3% crude fat, 11% crude fiber, a maximum
of 1.8% Ca, 0.62% P, 1.8% Na, and 1.2% K. The basal diet also contained
Cu sulfate, ferrous sulfate, zinc sulfate, and sodium molybdate plus
additional Cu in the form of the organic minerals described below. One
treatment contained 10 mg/kg of supplemental Cu as Cu proteinate (Cu
Bioplex®, Alltech, Nicholsville, KY); the second treatment contained
10 mg/kg of supplemental Cu as Cu amino acid complex (Cu Availamin®,
Zinpro, Eden Prarie, MN); and the third treatment contained 10 mg/kg
of supplemental Cu as Cu amino acid chelate (Cu MAAC®, Albion, Clearfield,
UT).
Each
source of Cu was thoroughly mixed with one third of the basal diet and
then each of the fortified basal feeds was pelleted by Carl S. Akey,
Inc. (Lewisburg, Ohio). Based on the total dry matter weight of the
feed, each treatment was calculated to contained 10 mg Mo/kg feed (sodium
molybdate), 0.25% S (from sulfates), and 500 mg Fe/kg feed (from sulfates).
Prior to feeding, each basal diet was assayed by plasma emission spectrophotometry
for Cu, Mo, S, and Fe.18, 19 The final analysis of each pelleted basal
diet indicated that it contained 20 mg Cu/kg feed, 10 mg Mo/kg feed,
0.35% S, and 750 mg Fe/kg feed. Each pelleted basal diet containing
a different source of Cu was identified by a different color feed tag
that matched the assigned color of the pen, as described above, so that
the correct basal diet was fed to the right group of animals.
The pelleted basal
diets were fed at the rate of 1.5 lbs (0.68 kg)/head/day. Additionally
the cattle were fed maize and free choice grass hay mixed with alfalfa
according to NRC recommendations at approximately the calculated levels
necessary to gain 3 lbs (1.36 kg)/day. All of the cattle were pen fed
morning and night and encouraged to eat at the same time. Maize and
hay intake were regulated to ensure complete consumption of the pelleted
basal diet.
Each of the animals received its assigned pelleted basal
diet daily for 120 days. At 60, 90, and 120 days of treatment, liver
biopsies and serum samples were obtained from each animal following
the procedures employed at initiation of the study. The liver samples
and half of the serum sample were assayed for Cu, Fe, and Mo. Ceruloplasmin
activity was concurrently determined from the other half of the serum
sample. At the conclusion of the trial at the time that the final liver
and serum samples were obtained, each animal was weighed again.
All of the collected
data were analyzed statistically using Systat® Version 10 (Systat Software,
Inc., Richmond, CA). Analysis of covariance (ANCOVA) was performed on
the individual time periods which accounted for and normalized the differences
in the means of groups and showed significant changes in the studied
variables in relation to their initial or previous variable values.
Multivariate analysis of covariance (MANCOVA) was also performed for
overall experimental error and to detect differences in the responses
of the studied variables based on previous variable values. By using
MANCOVA to determine experimental error and power univariant, ANCOVA
could be performed without increasing experimental error.
Results
The combined effects of Mo, S, and Fe significantly affected
Cu absorption in each group (p = 0.032) but these effects were greater
in the animals fed Cu proteinate or Cu amino acid complex sources compared
to those fed Cu amino acid chelate.
Table 1 presents the mean Cu, Fe, and Mo assays from the
liver biopsies at the initial phase of the study and at 60 days, 90
days, and 120 days of treatment. Below the actual numbers are the same
data expressed as percentages relative to the initial values. In the
initial phase to 60 day period, all of the treatments responded to the
dietary Cu antagonists (P < 0.001). As a percentage, by 90 days the
livers in the animals fed Cu amino acid chelate (amino acid chelate
group) contained significantly more Cu compared to either those fed
the Cu proteinate (proteinate group) or Cu amino acid complex (amino
acid complex group) (P < 0.005). The initial phase to 90 day declines
in the proteinate and the amino acid complex groups were 29.7% and 15.5%
greater, respectively, than the amino acid chelate group. From the initial
phase to 120 days the decline in the Cu content of the liver was 29.17%
more and 12.5% more for the proteinate and amino acid complex Cu sources
compared to the amino acid chelate sources. The differences between
the amino acid chelate and the proteinate or amino acid complex groups
were significant (P < 0.05). There was also a significant period
effect of the Cu antagonists on all of the groups from 90 days to 120
days compared to the 60 to 90 day period (P < 0.001).
Compared to the mean values at the commencement of the
study to 120 days, the Fe liver level in the proteinate group declined
the most (to 49% of initial). The amino acid chelate was next (to 64%
of initial) followed by the amino acid complex (to 77% of initial).
The decline in hepatic Fe in the proteinate group was significantly
greater than either the amino acid complex or amino acid chelate group
(P < 0.05).
There were no significant treatment or period effects
on the liver Mo values in any of the groups.
The ceruloplasmin activity for the 3 groups of steers
is presented in Table 2 with the same data being shown as percentages
of the initial ceruloplasmin activity below the actual values. During
the first 60 days of the study, there was a significant (P < 0.05)
period effect on each of the groups but no significant treatment effect.
The increased ceruloplasmin activity in the 3 groups from 60 to 90 days
was not significant. There was a significant change from the 90 to 120
day period compared to the 60 to 90 day period for all groups (P <
0.001). For the entire 120-day study, the increase in the ceruloplasmin
activity of the amino acid chelate group was significantly improved
(p = 0.086). The increases in the ceruloplasmin activity in the proteinate
and amino acid complex groups were not significant (P < 0.10).
Table
3 summarizes the mean quantities and changes in Cu, Fe, and Mo in the
serum of the 3 groups of animals. The same data are expressed as a percentage
relative to the initial measurements under the actual values. There
was no significant treatment effect on Cu serum values between the initial
phase and 60 days for any of the groups, but there was a significant
period effect on the Cu serum level of the proteinate group (P <
0.03). From 60 to 90 days there were significant treatment changes on
serum Cu in all 3 groups (P < 0.05). There were also significant
changes (P < 0.001) in the Cu serum values in all groups. There were
significant differences between the amino acid chelate group and the
other 2 groups (P < 0.08). At 90 to 120 days, there was a significant
(P < 0.08) increase in Cu serum levels for the amino acid chelate
group, whereas neither the proteinate nor the amino acid complex group
had significant increases in Cu serum levels.
For the Fe serum values from the initial phase to 120
days, the proteinate group increased 15.85%, the amino acid complex
group 19.19%, and the amino acid chelate group 26.49%. There was a significant
treatment difference between the 3 groups (P = 0.03) at the 60 day sampling
period, but by 90 days and beyond, there were no significant differences
in serum Fe.
In
the case of the Mo serum values, the proteinate group increased from
the initial phase to 60 days and then dropped from 90 days to 120 days
for a total increase from the initial phase to 120-day increase of 407%.
The mean Mo serum level of the amino acid complex group peaked at 60
days and then decreased. The total increase was 473% which was significantly
greater (P < 0.10) than when the study commenced. The mean serum
Mo level in the amino acid chelate group increased from the initial
phase to 60 days, decreased from 60 to 90 days, and then increased from
90 to 120 days. The total increase over the study period was 375%. The
differences in these percentage increases in each of the groups from
initiation of the study to 120 days were significant (P < 0.05).
The mean weight gain per animal per day was 2.5 lbs (1.14
kg). The weight gains between the groups were not significantly different.
Discussion
Given
the combined effect of the Cu antagonists in the experimental diets,
the levels of Cu administered were probably either too low or the levels
of inclusion of the antagonists too high to produce optimum Cu bioavailability
data. While bioavailability of all 3 sources of Cu appeared to be affected
by the total amounts of Mo, S, and Fe in the diet, this study indicated
that there are significant differences in the degree of Cu suppression
depending on the source of supplemental Cu. Since each manufacturer
of the Cu sources claims to produce its product differently, the differences
seen in this study possibly originate in those manufacturing methods.
Despite the observed differences in the cattle’s responses
to the three sources of organic Cu, a future study should consider either
increasing the Cu levels in each group or reducing the levels of the
Cu antagonists. A greater biological response to the Cu supplements
would have probably resulted if either of these changes had been made.
Based on previous observations, the combined effect of
Mo, S, and Fe on Cu absorption and metabolism was fairly predictable.1–6
Nevertheless, a future study should perhaps include a control group
of animals that received no supplemental Cu.
Initially, the Cu proteinate appeared to be the most strongly
affected by the antagonists, suggesting a possibility of a greater ruminal
hydrolyzation of the molecule and subsequent Cu ionization due to low
chelate stability. While there was slightly more hepatic Cu in this
group at study initiation, as a function of both time and treatment,
it declined the greatest suggesting the potential for further decline
had the study continued past the 120 days. Cu is delivered to the liver
via the serum, and the final serum Cu of the proteinate group was significantly
less than that of the amino acid chelate group. Since the liver is responsible
for the production of ceruloplasmin using serum-delivered Cu, one explanation
for the lower ceruloplasmin activity of the proteinate group is lower
Cu serum values and larger hepatic Cu level decline compared to the
amino acid chelate group. This suggests that the dietary Cu antagonists
had a greater effect on the proteinate source.
The Cu amino acid complex group had numerically higher
Cu liver, serum, and ceruloplasmin values than the Cu proteinate, but
all of these measurements were not statistically different. This suggests
that the dietary Cu antagonists introduced into the feed of the group
receiving the amino acid complex had about the same effect on this group’s
Cu status as the antagonists had on the group fed with the Cu proteinate.
This leads to the conclusion that
Cu proteinate and
Cu amino acid complex may be equivalent sources of dietary Cu.
The
Cu amino acid chelate was the only supplemental source of Cu that demonstrated
significant resistance to the Cu antagonists. The amino acid chelate
group had the least total decline in hepatic Cu, thus suggesting that
more Cu from the amino acid chelate was absorbed and metabolized than
from either the proteinate or complex groups. Additional absorption
studies are indicated to determine the exact percentage absorbed or
inhibited.
The amino acid chelate group was the only group showing
a significant increase in ceruloplasmin activity from initiation to
120 days, suggesting that, as Cu antagonists built up in the body over
time, only the Cu amino acid chelate source was able to overcome these
deleterious effects. The data suggest that more Cu was transported to
the tissues from the amino acid chelate source compared to either the
Cu proteinate or Cu amino acid complex sources. These observations are
borne out by the significantly higher serum Cu levels at 90 to 120 days
in the amino acid chelate group. It is interesting to note that, following
the initial decline in Cu status of the amino acid chelate group, it
commenced correcting itself after the 60-day period. Statistically,
neither the proteinate group nor the amino acid complex group followed
the same pattern. Had the experiment not been terminated at 120 days,
a more normal Cu status may have ultimately resulted in the amino acid
chelate group. This perhaps should be the subject of an additional longer-term
study.
There was a smaller increase in the Mo serum levels of
the amino acid chelate group compared to the proteinate and amino acid
complex groups. The potential effects of Mo on Cu levels in the blood
are summarized in Figure 1. Absorbed MoS42– can either increase the binding capacity of the ceruloplasmin
and reduce tissue levels of Cu, or it can directly bind with plasma
Cu and reduce ceruloplasmin activity. Because of the total increases
in ceruloplasmin activity in all 3 groups coupled with reduced hepatic
Cu level, the absorbed Mo appeared to influence the binding capacity
of the ceruloplasmin. The amino acid chelate group appeared to be the
least affected suggesting some of the copper may have remained chelated
to the original amino acids even after absorption. If that were the
case, it would confirm certain other absorption studies.7 This possibility
needs further investigation.
It is also possible that the increased serum Mo complexed
more serum Cu resulting in decreased ceruloplasmin activity in both
the proteinate and amino acid complex groups compared to the amino acid
chelate group. If that were the case, it would suggest that at least
some of the amino acid chelate derived Cu arrived in the blood in a
more stable (less reactive) form than either the proteinate or amino
acid complex sources of Cu. These observations indicate the need for
more studies.
Why more Mo was absorbed by the proteinate and amino acid
complex groups compared to the amino acid chelate group is unknown and
should also perhaps be the subject of further study. All 3 groups of
steers had less hepatic Fe at the conclusion of the study compared to
commencement levels. This is surprising since supplemental Fe was fed.
In view of the serum Fe increases, perhaps Fe secretion occurred. Another
explanation may be that iron ions are bonding with the sulfhydryl groups
on the Cu transport proteins to form insoluble complexes, thus reducing
the actual absorption of both Fe and Cu. A third possibility is that
the dietary Fe was poorly absorbed for some other reason, causing increased
mobilization of liver Fe. More research would be required to elucidate
these observations.
A future study should perhaps consider which source of
organic Cu was able to most rapidly restore Cu status to the Cu depleted
steers. A future study should also incorporate other research parameters
that would provide additional and more definitive data on the effects
of the different Cu sources on cattle.
Finally,
the daily weight gains were not as great as the formulated diet projected.
Perhaps this was due to inadequate available Cu to support necessary
growth and underscores the importance of available Cu in the diet of
growing cattle. Another explanation lies in the research design. It
was more important to limit the quantity of free choice feed to ensure
that the cattle would consume all of the basal diets containing the
treatments than it was to optimize gain. In managing feed consumption,
gain potential may have been affected.
Conclusions
Although none of the 3 organic sources of Cu could
maintain the pre-trial Cu tissue and serum values, there were significant
differences in metabolic responses based the Cu source. The amino acid
chelate source of Cu appeared to overcome effects of the Cu antagonists
more effectively than either the Cu proteinate or the Cu amino acid
complex and provided more available Cu to the animals. Based on this
study, it was concluded that Cu amino acid chelate was more bioavailable
than either the Cu proteinate or Cu amino acid complex and thus produced
a significantly greater positive metabolic response.
References
1. Suttle NF, Field AC: Effects of dietary supplements
of thiomolybdates on copper and molybdenum metabolism in sheep” J Comp
Path 93:379–389, 1983.
2. Suttle NF, Abrahams P, Thornton I: The role of
a soil x dietary sulphur interaction in the impairment of copper absorption
by ingested soil in sheep. J Agri Sci Camb 103: 81–86, 1984.
3. Mills CF: Metabolic interactions of copper with
other trace elements in Mills CF, ed. Biological Roles of Copper. Amsterdam:
Excerpta Medica; 46, 1980.
4. Mills CF,
Bremner I, El-Gallad TT, et al: Mechanisms of the Mo/sulphur antagonism
of copper utilization by ruminants in Kirchgessner, M, ed. Trace Elements
in Man and Animals – 3 Freising: Institut Für Ernährungsphysiologie;
150–158, 1978.
5. Humphries WR, Phillipo M, Young BW, Bremner I:
The influence of dietary Fe and Mo on copper metabolism in calves. Br
J Nutr 49:77–86, 1983.
6. Suttle NF, Peter DW: Rumen sulphide metabolism
as a major determinant of copper availability in the diets of sheep.
in Mills CF, Bremner I, Chesters JK, eds: Trace Elements in Man and
Animals – 5 Farnham: Royal Slough Commonwealth Agricultural Bureau;
367–370, 1985.
7. Ashmead HD, Graff DJ, Ashmead HH: Intestinal Absorption
of Metal Ions and Chelates. Springfield: Charles C. Thomas; 71–102,
1985.
8. Ashmead H: Tissue transportation of organic trace
minerals. J Appl Nutr 22:42–51, 1970.
9. Mason K: A conspectus of research on copper metabolism
and requirements of man. J Nutr 109:1990, 1979.
10. Bachman PM, ed: Official Publication Association
of American Feed Control Officials Incorporated Oxford: AAFCO; 278,
2003.
11. Kratzer FH, Vohra P: Chelates in Nutrition. Boca
Raton: CRC Press; 5–27, 1986.
12. Power R, Horgan K: Biological chemistry and absorption
of inorganic and organic trace metals. Feed Compounder 21:25–31, 2001.
13. Wapnir R: Protein Nutrition and Mineral Absorption.
Boca Raton: CRC Press; 1990.
14.
Bovell-Benjamin AC, Viteri F, Allen LH: Fe absorption from
ferrous bisglycinate and ferric trisglycinate in whole maize is regulated
by Fe status. Am J Clin Nutr 71:1563–15699, 2000.
15.
Ashmead HD: The absorption and metabolism of iron amino
acid chelate. Arc Lat Am J Nutr 51(Supp 1):13, 2001.
16.
Hickok D, Carlson M, Brink D, et al: Multi-elemental analysis
of bovine liver biopsy and whole liver. in Brink D, ed: University of
Nebraska 1996 Beef Cattle Report. Lincoln: University of Nebraska; 1996.
17.
USEPA: Test methods for evaluating solid waste: physical/chemical
methods; Final Update III Method. 3050B, USEPA SW-846, 1997.
18.
USEPA: Test methods for evaluating solid waste: physical/chemical
methods; Final Update III Method 6010B, USEPA SW-846 , 1997.
19.
Rice E: Standardization of ceruloplasmin activity in terms of
International Enzyme Units. Anal Biochem 3:452–456, 1962.
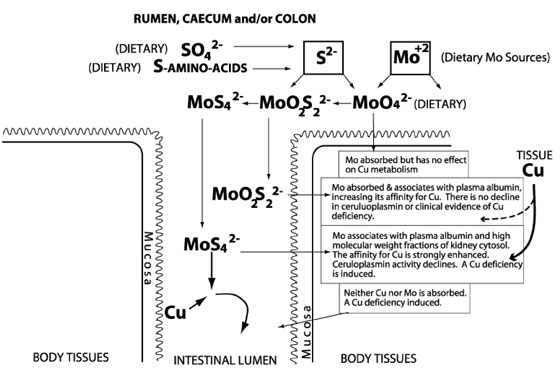
Figure 1. The
effect of Mo and S complexes on Cu absorption and metabolism. Reprinted
from Mills;3 with permission.
Table
1. Mean Cu, Fe, and Mo Contents
of Liver
Group Initial 60 Days 90 Days 120
Days
Copper Proteinate 208.33 ± 83.472 82.30 ± 46.67* 49.11 ± 24.31 35.59
± 19.73†
100.0% 39.5% 23.6%
17.5%
Amino acid complex 205.56 ± 40.09 87.81 ± 27.99* 54.59 ± 20.83 43.66 ± 20.67†
100.0% 42.7% 26.5%
21.5%
Amino acid chelate 174.34 ± 54.18 62.59 ± 26.64* 53.38 ± 26.08‡ 41.32 ± 24.35†§
100.0% 35.9% 30.6% 23.7%
Iron
Proteinate 435.22 ± 182.0 329.00 ± 161.49
222.78 ± 69.00 212.44
± 38.10
100.0% 75.6% 51.1%
48.8%
Amino acid complex 321.44 ± 87.62 197.38 ± 47.74 198.56 ± 57.23 247.89 ± 123.79§
100.0% 61.4% 61.7%
77.1%
Amino acid chelate 328.11 ± 91.00 236.63 ± 99.19 227.78 ± 63.68
208.67 ± 39.9§
100.0% 71.9% 69.4%
763.5%
Molybdenum
Proteinate 3.04 ± 2.07 4.67 ± 1.52
4.11 ± 1.19 2.91
± 0.63
100.0% 153.0% 135.0%
95.7%
Amino acid complex 3.03 ± 0.58 4.23 ± 1.17 4.39
± 0.79 3.46 ± 0.98
100.0% 139.0% 145.0% 114.0%
Amino acid chelate 2.89 ± 0.45 3.71 ± 0.88 4.72
± 0.64 3.46 ± 0.30
100.0% 128.0% 163.0% 120.0%
Table
2. Mean
Ceruloplasmin Activity
Group Initial 60 Days 90 Days 120 Days
Proteinate 42.22 100.0% 46.22* 109.0%
48.11 114.0% 52.67† 124.0%
Amino
acid complex 42.44 100.0% 47.78* 113.0%
60.44 142.0% 58.11† 137.0%
Amino
acid chelate 42.44 100.0% 51.67* 122.0%
49.78 117.0% 61.44†‡ 144.0%
Table
3. Mean Changes in Cu, Fe, and
Mo in Serum
Group Initial 60 Days 90 Days 120
Days
Copper Proteinate 0.84
0.63* 0.61†‡
0.60
100.0% 75.0% 72.6%
71.4%
Amino acid complex 0.76 0.67
0.66†v
0.70
100.0% 88.0% 86.8% 92.1
Amino acid chelate 0.84 0.73
0.63†‡
0.74§
100.0% 86.9% 75.0% 88.1%
Iron
Proteinate 1.83 2.51§ 2.41 2.12
100.0% 137.1% 131.7% 115.8%
Amino acid complex 1.72 2.83§ 2.10 2.05
100.0% 164.5% 122.1% 119.1%
Amino acid chelate 1.51 2.35
2.23 1.91
100.0% 155.6% 147.7% 126.5%
Molybdenum
Proteinate 0.014 0.166 0.067 0.057**
100.0% 118.5% 478.0% 407.0%
Amino acid complex 0.015 0.155
0.067 0.071**
100.0% 103.3% 446.0% 473.0%
Amino acid chelate 0.016 0.054
0.053 0.060**
100.0% 33.7% 331.0% 375.0%